Electrically Evoked
Otoacoustic Emissions
Jiefu Zheng, Yuan Zou
NOTE : This
editorial is also available as a PDF
file
1. Introduction
Electrically evoked otoacoustic emissions
(EEOAEs) are acoustic signals emitted from the cochlea to the external ear
canal when alternating current is delivered into the cochlea (Hubbard and Mountain,
1983; Mountain and Hubbard, 1989; Murata et al., 1991; Ren and Nuttall, 1995). Unlike acoustically
evoked OAEs, the EEOAEs are considered ?non-natural? because the cochlea is
under the artificial situation stimulated with electrical currents rather
than sounds. In addition, due to the need to open the bulla and place stimulation
electrodes into/onto the cochlea, the procedures for EEOAE measurement are
invasive. In general, there are two ways to apply current injection, one being
the intracochlear and the other being the extracochlear stimulation. To our
knowledge, the published reports of EEOAE measurements were conducted on animals.
Animal species used for EEOAE research include gerbil, guinea pigs, chinchilla,
lizards and chicken (Hubbard and Mountain, 1983; Nuttall and Ren,
1995; Sun et al., 2000; Manley 2001; Chen et al., 2001). The EEOAEs can always
be obtained in normal ears.
The EEOAEs occur at the
same frequency as that of the electrical stimulus as a result of
fast electromotile responses of the outer hair cells (OHCs) that
underlie the reverse transduction process (i.e., electrical-to mechanical
transduction) (Mountain and Hubbard,
1989; Nuttall and Ren, 1995). They are reduced or absent in animals in which OHCs are
damaged after treatment with ototoxic drugs or by acoustic injury
(Ren and Nuttall, 2000;
Reyes et al., 2001; Zheng et al., 2001; Nakajima et al., 1996). Experiments on basilar
membrane (BM) motion induced by electrical stimulation indicate
that OHCs undergoing electrically evoked motion are capable of producing
high-fidelity, high frequency mechanical energy. The electrically
evoked intracochlear energy results in conventional traveling waves
within the cochlea, as well as emissions of sound from the cochlea
(Xue
et al., 1995; Nuttall and Ren, 1995). It is a consensus that the EEOAEs are generated
at the location near the stimulation electrode. However, the mechanisms
of EEOAE generation differ with methods for current delivery. It
is thought that electrical currents delivered to the scala media
(SM) pass through the transduction channels. Thus the EEOAEs are
generated or modulated by mechanisms related
to cochlear transduction (Yates and Kirk, 1998). In contrast, current delivered into the scala
tympani (ST) (directly or from round window stimulation) may extracellularly
stimulate the OHCs by directly affecting the transmembrane voltage
of OHCs, resulting in voltage-dependent OHC motion (Ren and Nuttall, 1995;
Nuttall and Ren, 1995; Nuttall et al., 2001).
SM-applied current is thought to stimulate
a spatially well-defined population of OHCs due to the confining
effect of the SM space constant, which is estimated to be 1.5 to
4 mm (Xue et al., 1993; Nakajima
et al., 1994). The space constant is frequency-dependent and as such it could
be significantly shorter than 1.5 mm in the cochlear basal turn.
The magnitude transfer functions (i.e., the magnitude of emissions
as a function of the frequency) are relatively narrow band or low-pass
functions and the slope of the phase-versus-frequency function of
the emission (the group delay) is related to the tonotopic location
of the SM electrode (Murata et al., 1991;
Nakajima et al., 1994; Kirk and Yates, 1996) (see Figures 1 and 2). Similarly, currents
applied in the ST result in emissions with tonotopically organized
band-pass bandwidth properties as well (Nuttall et al., 2001) (Figure 3). In contrast,
EEOAE transfer functions from passing current onto the round window
(RW) are considerably broader in frequency (being from 60 Hz to
50 kHz in guinea pigs) with a group delay apparently consistent
with the expected reverse propagation time from cochlear locations
near the round window (Ren and Nuttall, 1995;
Nuttall et al., 2001) (Figure 3).
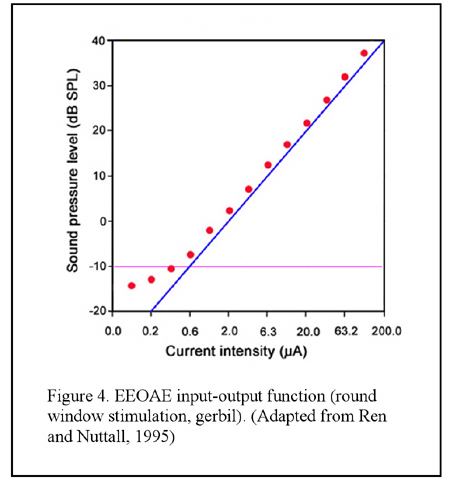
When
sweeping the electrical current with small frequency steps the resultant
EEOAE transfer function shows peaks and notches in magnitude, the
appearance being termed as ?fine structure?, which is similar to
that has been observed in the DPOAE gram (Figure 3). Unlike the
acoustically evoked OAEs, the EEOAE input-output function is generally
linear even in sensitive cochlea (Figure 4), though nonlinearity
could be observed in certain conditions (Ren and Nuttall, 1995;
Nakajima et al., 1998). Nevertheless, simultaneously applied electrical currents
at two frequencies (f1 and f2) are able to evoke DPOAEs as what
are evoked by acoustic primaries (Ren et al., 1996)
EEOAEs can be modulated by an acoustic stimulus. It
was firstly reported by Mountain and Hubbard and then by others that a simultaneously
presented sound could enhance the EEOAEs evoked by currents applied into the
SM (Mountain and Hubbard, 1989; Xue et al., 1993; Nakajima et al.,
1994; Kirk and Yates, 1996) (see Figure 1). The enhancement is largest when the acoustic
frequencies are near the characteristic frequency (CF) of the current injection
location (Xue, et al., 1993). This enhancement is hypothesized as being the
result of the opening of the negative feedback loop of the cochlear amplification
by acoustic stimulation that causes reduction of the forward transduction.
The ?opening? of the feedback loop, in this hypothesis, removes the suppression
of the mechanical response and consequently results in an increased amplitude
of the EEOAEs (Mountain and Hubbard, 1989). However, data inconsistent
with this hypothesis were reported by others (Kirk and Yates, 1996). In round
window evoked EEOAE study, it was observed that a high sound level acoustical
tone enhanced the EEOAE fine structure at frequencies below that of the acoustical
stimulus, and suppressed the overall level of the EEOAEs at frequencies above
the acoustical frequency. The acoustic modulations on the EEOAEs was most
efficient for frequencies approximately one half octave lower than the acoustical
frequencies (Ren and Nuttall, 1998). The underlying mechanisms
for these modulations were hypothesized to be the interaction between the
electrically and acoustically evoked basilar membrane motions and/or the acoustical
stimulus induced basilar membrane impedance discontinuity at its CF location
that leads to a change of the electrically evoked traveling waves (Ren
and Nuttall, 1998).
There is increasing evidence in favor of the hypothesis that
the EEOAEs recorded in the ear canal have more than one origin on
the BM. By using multiple component analysis method, a long delay
component (LDC) and a short delay component (SDC) of EEOAEs were
identified (Ren and Nuttall, 2000;
Zou et al, 2003) (Figure 5). By observing the effects of furosemide, quinine, and
other pathophysiological cochlear conditions on the multiple components
of EEOAEs (Ren and Nuttall, 1998,
2000; Zheng et al, 2001), it was found that the LDC of the EEOAEs is
closely related to the cochlear sensitivity and is vulnerable to
cochlear damage. It was also found that the fine structure of EEOAEs
was a feature of sensitive cochlea and depended on the presence
of the LDC. The fine structure is postulated to result from the
cancellation/enhancement effects of multiple sound waves in healthy
cochlea. In contrast, the overall magnitude of EEOAEs is mainly
related to the SDC and is relatively less sensitive to cochlear damage. Therefore, it is hypothesized
thatelectrical currents delivered
into the cochlea result in OHC motion and hence the basilar membrane
vibration at the site near the stimulation electrode, giving rise
to energy propagation to both forward (to apical) and backward (to
basal) directions. The energy propagating backwards to the oval
window results in stapes vibration, giving rise to the SDC. Whereas,
the energy propagating forward will reflect from its own CF location,
forming the LDC (Ren and Nuttall, 2000; Zou et al, 2003) (Figure 6).
2. Methods of EEOAE measurement
Experimental animals need to be anesthetized. A microphone
is coupled to the ear canal to measure the acoustic signal generated in the
cochlea by the electrical stimulation. In order to expose the cochlea for
placement of the stimulation electrodes the bulla needs to be opened. The
middle ear muscle tendons should be sectioned to avoid electrically evoked
muscle contractions. The stimuli are usually sinusoidal constant currents
delivered through an optically-isolated constant-current source. The acoustic
signals in the ear canal are recorded in terms of magnitude and phase at the
frequency of the electrical current.
Two approaches for electrical current stimulation are used
to evoke the OAEs: (1) Intracochlear stimulation. Holes are made in the
cochlea and electrodes are placed into the cochlea to deliver the current
stimuli. According to the location of the electrode placement, there are two
types of intracochlear stimulation, the scala media stimulation and scala tympani stimulation. (2) Extracochlear stimulation. The electrode is placed
in the round window niche so that the current is delivered through the round
window membrane into the scala tympani. The cochlea is kept intact in this
situation.
Scala media stimulation: A glass microelectrode is inserted into the scala media through the
lateral wall or the basilar membrane (Figure 7). Constant current
is injected into the scala media using the microelectrode. The tip
diameter of the electrode is approximately 5 mm and the microelectrode
is filled with 0.16 M, 1 M, or 3 M KCl. A Ag/AgCl wire is inserted
into the neck muscles to serve as the current return electrode.
The intensity of the currents could range from 1 to 50 mA peak-to-peak.
Scala tympani stimulation:
A platinum-iridium wire is usually used (50-75 mm in diameter) for electrical current stimulation. The electrode is
placed into the scala tympani (Figure 8). For monopolar electrode
configuration, the Ag/AgCl wire (serving as the return electrode)
is inserted into the neck muscles. For bipolar electrode configuration,
a second platinum-iridium wire is placed into the scala tympani
(or scala vestibuli) as the return electrode. The intensity of the
currents could be 10 to 50 mA rms.
Extracochlear (round
window) stimulation: The electric current is delivered to the scala tympani through a platinum-iridium
electrode in the round window niche. The Ag/AgCl wire in the neck
serves as the return electrode (Figure 9). The electric current
level could be from 10 to 300 mA rms.
3.
Data analysis and presentation
?
Magnitude and phase transfer
functions
Magnitude and phase are essential information for EEOAEs research.
The magnitude transfer function curve is obtained by plotting the magnitude
as a function of the frequency, which provides information of the frequency
response or bandwidth features (Figure 1 and Figure 3). As that has been mentioned
in the Introduction, the EEOAE magnitude transfer function presents a feature
of ?fine structure? in sensitive cochlea. While the overall level of the EEOAEs
rises as the current intensity increase, the amplitude of fine structure (the
peaks and notches in magnitude transfer function) tends to decrease with the
increasing mean EEOAE level. When the phase (in degree or radian) is plotted
against the frequency the phase transfer function curve will be obtained in
that group delay could be calculated from the slope (see Figure 2 and Figure
3).
?
Input-output function
By plotting the magnitude of EEOAEs as a function of the electrical
current intensity the input-output function (I/O function) is obtained. The
I/O function of the EEOAEs is generally linear (see Figure 4).
?
Multiple component analysis
The presence of the fine structure suggests the existence of multiple
sources of the EEOAEs and consequently, multiple components of the recorded
emissions. A method of multiple component analysis (MCA) has been developed
(Ren and Nuttall, 2000) in that the real part of the emission is calculated
from the magnitude and phase spectra and then the multiple components
are extracted. By using the MCA for EEOAE data analysis, long delay component
(LDC) and short delay component (SDC) are observed (Figure 5 and Figure 10).
4. Hearing sensitivity and EEOAEs
It has been demonstrated that the OHCs are the origin of the
EEOAEs. Thus the EEOAEs provide a tool for the study of in vivo OHC electromotility. The
magnitude and the fine structure (in the magnitude-frequency transfer function)
of the EEOAEs are associated with the cochlear sensitivity. Any kind of insult
to the cochlea that inhibits the OHC function will result in EEOAEs fine structure
diminution and/or magnitude reduction. The fine structure is a feature of
the sensitive cochlea and is very vulnerable to any damage on the OHCs whereas
the overall mean magnitude of EEOAEs is relatively less sensitive to the damage.
In fact, the reduction of the EEOAE magnitude is not proportional to the cochlear
sensitivity loss. Even in the case when the animal is dead and the fine structure
is abolished, the overall magnitude of EEOAEs is reduced by only about 15-20
dB, and residual EEOAEs still can be observed. Moreover, total loss of hair
cells further reduces the EEOAE magnitude but generally residual emissions
are still seen.
References:
Chen L, Sun W, Salvi RJ., Electrically
evoked otoacoustic emissions from the chicken ear. Hear Res. 2001
Nov;161(1-2):54-64.
Hubbard, A.E., Mountain, D.C., 1983. Alternating
current delivered into the scala media alters sound pressure at
the eardrum. Science, 222, 510-512.
Kirk, D.L., Yates, G.K., 1996. Frequency tuning
and acoustic enhancement of electrically evoked otoacoustic emissions
in the guinea pig cochlea. J. Acoust. Soc. Am. 100, 3714-3725.
Mountain, D.C., Hubbard, A.E., 1989. Rapid force
production in the cochlea. Hear. Res. 42, 195-202.
Manley, GA., 2001. Evidence for an active
process and a cochlear amplifier in nonmammals. J. Neurophysiol.
86(2), 541-9. (Review)
Murata, K., Moriyama, T., Hosokawa, Y., Minami, S.,
1991. Alternating current induced otoacoustic emissions in the guinea
pig. Hear. Res. 55, 201-214.
Nakajima, H.H. and Olson, E.S., 1994. Electrically
evoked otoacoustic emissions from the apical turns of the gerbil
cochlea. J.Acoust. Soc. Am. 96 (2), 786-794.
Nakajima, H.H., Olson, E.S., Mountain, D.C., and Bubbard
A.E., 1996. Acoustic overstimulation enhances low-frequency
electrically-evoked otoacoustic emissions and reduces high-frequency
emissions. Auditory Neuroscience. 3, 79-99.
Nuttall, A.L., Ren, T., 1995. Electromotile hearing:
evidence from basilar membrane motion and otoacoustic emissions.
Hear. Res. 92, 170-177.
Nuttall, A.L., Zheng, J., Ren, T., de Bore, E.,
2001. Electrically evoked otoacoustic emissions from apical and
basal perilymphatic electrode positions in the guinea pig cochlea.
Hear. Res. 152, 77-89.
Ren, T., Nuttall, A.L., 1995. Extracochlear electrically
evoked otoacoustic emissions: a model for in vivo assessment of
outer hair cell electromotility. Hear. Res. 92, 178-183.
Ren, T., Nuttall, A.L., Miller, J.M., 1996. Electrically
evoked cubic distortion product otoacoustic emissions from gerbil
cochlea. Hear. Res. 102 (1-2), 43-50.
Ren, T., Nuttall, A.L., 1998. Acoustic modulation
of electrically evoked otoacoustic emission in intact gerbil cochlea.
Hear. Res. 120, 7-16.
Ren, T., Nuttall, A.L., 2000.
Fine structure and multicomponents of the electrically evoked otoacoustic
emission in gerbil. Hear. Res. 143, 58-68.
Reyes S, Ding D, Sun W, Salvi R., 2001. Effect of
inner and outer hair cell lesions on electrically evoked otoacoustic
emissions. Hear Res. 158, 139-50.
Sun, W., Ding, D., Reyes. S., Salvi R.J., 2000. Effects
of AC and DC stimulation on chinchilla SOAE amplitude and frequency.
Hear Res. 150, 137-148.
Xue, S., Mountain, D.C., Hubbard, A.E., 1993. Acoustic
enhancement of electrically-evoked otoacoustic emissions reflects
basilar membrane tuning: Experiment results. Hear. Res. 70, 121-126.
Xue, S., Mountain, D.C., Hubbard, A.E., 1995. Electrically
evoked basilar membrane motion. J. Acoust. Soc. Am. 97, 3030-3041.
Yates G.K. and Kirk D.L., 1998. Cochlear electrically
evoked emissions modulated by mechanical transduction channels.
J. Neurosci. 18 (6), 1996-2003.
Zheng, J., Ren, T., Parthasarathi, A., Nuttall, A.L.,
2001. Quinine induced alterations of electrically evoked otoacoustic
emissions and cochlear potentials in guinea pigs. Hear. Res. 154,
124-134.
Zou Y. Zheng, J., Nuttall, A.L., Ren, T., 2003.
The sources of electrically evoked otoacoustic emissions, Hear.
Res. 180, 91-100